Reseach projects
Facing targets sputtering (FTS) technique
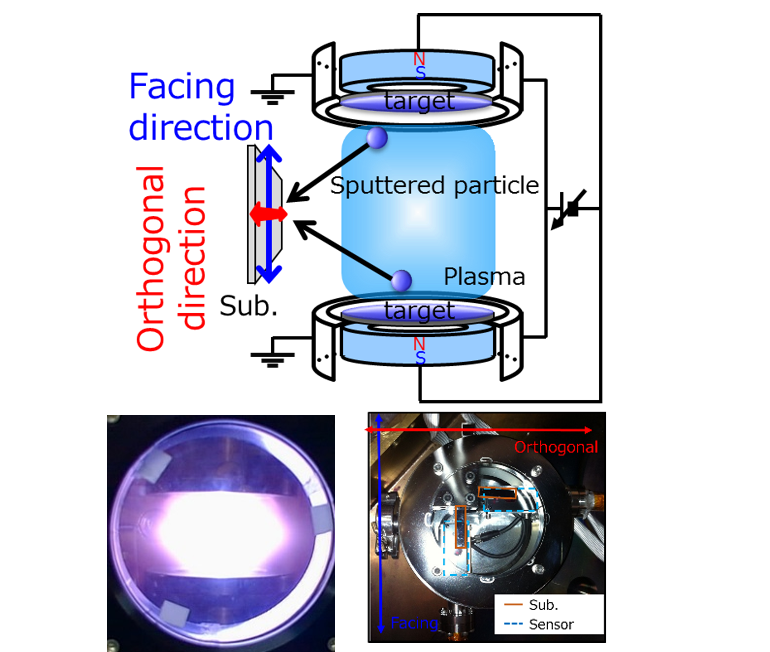
Our group has odeveloped a facing targets sputtering technique, plasma-damge free sputtering, as an original thin film fabrication technique.
Two targets facing each other are placed and used as cathode electrodes.
Magenetic filed is applied perpendicularly to the targets surfaces to conflict high-density plasma in the bottom of the targets.
Recently, we extensively use the FTS system to research and develop new functional spin materials and permanent magnet thin films.
In addition, anomolous stress in sputtered films can be yieleded by FTS.
As futher studies, highly sensitive in-situ stress observation system was built in our FTS system.
Stress induced in emerging magnetic recording thin films has been characteriezed.
Development of next-generation information storage and memory
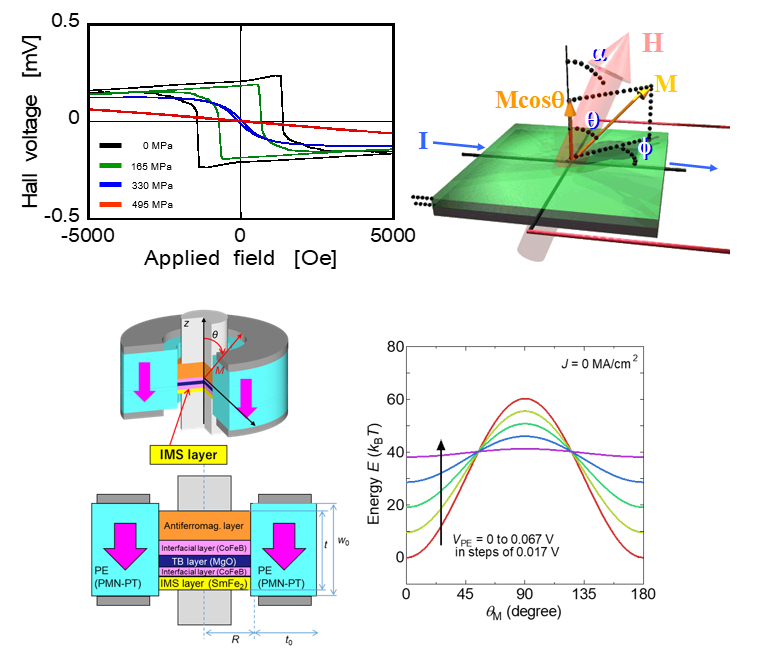
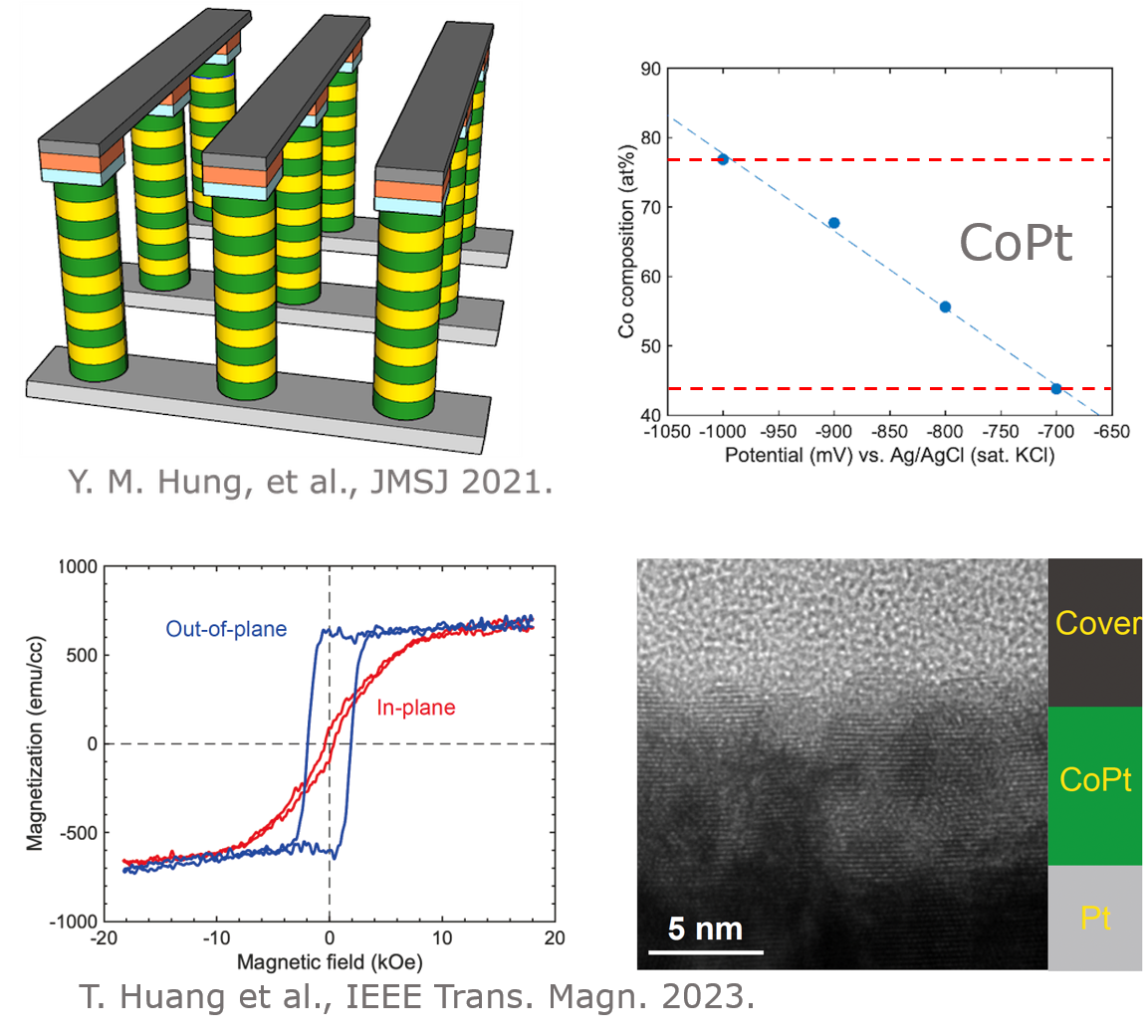
Strain-assisted magnetization reversal and piezo-electronic magnetoresistive device
MRAM consumes a great deal of power during magnetization reversal operations, so the power reduction effect of nonvolatility is still limited. In addition, reducing the energy barrier to magnetization reversal in order to lower the power consumption will in turn reduce the magnetization's resistance to thermal perturbation. Therefore, our laboratory has devised stress-assisted inversion magnetization reversal (SAMR), in which the energy barrier is temporarily reduced during magnetization reversal only by utilizing the piezoelectric and inverse magnetostrictive effects. In collaboration with other laboratories, we have proposed a piezo-electronic magnetoresistive device structure that can be integrated with highly efficient pressure application. We are also developing perpendicularly magnetized super magnetostrictive materials for this device and evaluating the power consumption reduction effect of SAMR using simulations.
Ultra-low-loss nonvolatile memory based on the interaction between superconductivity and spin
Ferromagnetism is a property of spin alignment, whereas the Cooper pair of superconductivity consists of electrons with opposite spin orientations. When two materials with such opposing properties are joined, very interesting phenomena occur at the interface. Such phenomena can be industrially exploited, and especially with the recent development of quantum computer technology, it has become realistic to operate electronic devices at very low temperatures. In Nakagawa's laboratory, we are aiming to realize ultra-low-loss memory by utilizing the lossless nature of superconductivity and the nonvolatility of spin. We are also developing the evaluation of the spin polarization rate of conduction electrons in spintronic materials by utilizing the phenomena occurring at the interface between superconductivity and ferromagnetism.
Development of magnetic materials for 3D magnetic memory
Today's information society is supported by information storage technologies such as SSD (Solid-state disc) and HDD (Hard disk drive). As the amount of information produced by human beings will increase exponentially in the future, there is a need to develop information storage technologies that can operate at higher speeds and with larger capacities. Currently, in the JST-CREST project, research and development of 3D magnetic memory as a new information storage memory is being conducted, in which Nakagawa Lab. is also participating.
This memory is based on a recently discovered phenomenon in spintronics: current-driven magnetic wall movement, in which the S/N boundary moves when an electric current is applied to a nano-sized magnet. In a cassette tape, which is no longer likely to be used by today's students, the S/N direction of the magnetization of tiny magnetic particles applied on the tape was moved to the top of the reading sensor by moving the tape, and the magnetic information was read one after another. This 3D magnetic memory is a "magnetic tape without any driving parts. Nakagawa laboratory has been studying spintronics thin films using the electrolytic plating method, which is considered to be the only method to fabricate the magnetic layer of this 3D magnetic memory, by taking advantage of the accumulation of magnetic thin films accumulated so far.
Development of next generation power magnetics materials
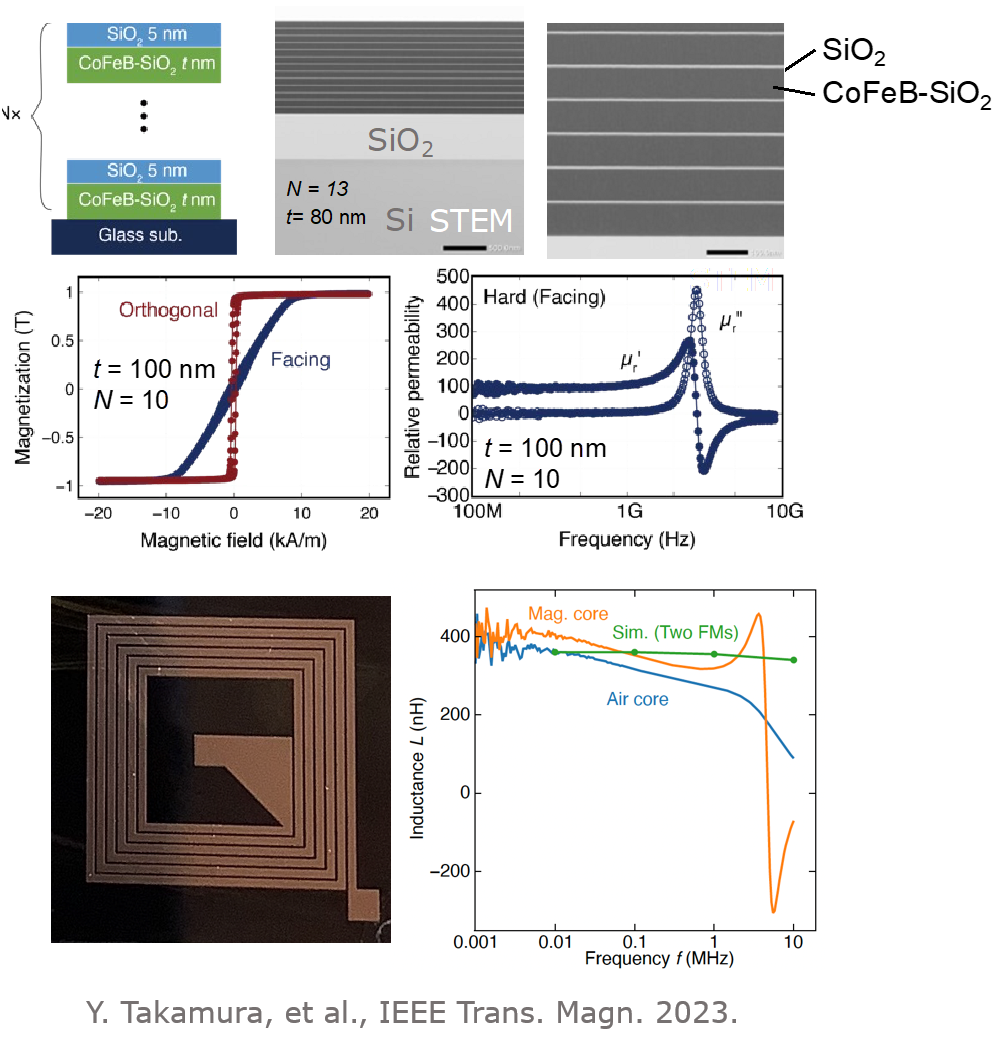
Nanogranular thin films for high-frequency power magnetics
Power electronic circuits have been improved in performance and miniaturization by increasing the switching frequency. This shift to higher frequencies has been dramatically accelerated by the emergence of wide-gap semiconductors such as SiC and GaN. Currently, the bottleneck of high frequency has become the soft magnetic materials used in inductors, and the development of new materials is an urgent issue.
Our laboratory is conducting research and development of nano-granular materials fabricated by the opposed-target sputtering method as magnetic materials used in inductors fabricated by integrated circuit technology, which is advantageous for high-frequency operation. Nano-granular materials are materials in which nano-sized magnetic particles are dispersed in an electric insulator, and have low loss due to eddy currents even when operated at high frequencies. We have achieved nanogranular thin films with excellent soft magnetic properties by imparting large induced magnetic anisotropy using the opposed-target sputtering method.
Development of Integrated Inductors for PwrSoC
We are conducting research on high-frequency inductors using semiconductor fabrication processes for PwrSoC (Power Supply on Chip), which realizes all power electronics circuits in a single chip format. We have introduced a nanogranular thin film formed by facing target sputtering and are investigating its effect on device characteristics.
Next generation thermoelectric power generation material thin film
Exploration of Fe-based Heusler alloys using the RTA alloying method
Heusler alloy thin films have long been studied as spintronics materials in Nakagawa Laboratory. Recently, Fe-based Heusler alloy thin films have been attracting attention as a material with excellent thermoelectric performance, and we are aiming to apply the technology of ultra-high quality Heusler alloy thin films of the same quality as the bulk, such as the Rapid thermal alloying method, to Fe-based Heusler alloy thin films to form even higher performance thermoelectric Heusler alloys with even higher performance. In collaboration with other laboratories, we are conducting research with an eye toward device realization (i.e., thermoelectric power generation devices).